Updated One Earth Climate Model (OECM): Decarbonizing all sectors of the economy to limit global temperature rise to 1.5°C
- Renewable Energy
- Renewable Power
- Renewable Heat
- Renewable Transport
- Energy Efficiency
- Sustainable Biomass
- Offshore Wind
- Onshore Wind
- Solar Thermoelectric
- Geothermal
- Wave Energy
- Green Hydrogen
- Solar Heat
- District Heat
- Electric Heat
- Sustainable Synfuel
- Electric Transport
- Transportation Efficiency
- Science & Technology
In the past few years, a growing number of countries and companies have pledged to reach net-zero emissions by 2050 in line with The Paris Agreement. Despite this growth, there is still little consensus on how these targets will be reached, with major inconsistencies in the speed and breadth of emissions reductions. According to the IPCC, future global emissions cannot exceed 400 billion tons of CO2 from 2020 on if we are to limit temperature rise to 1.5˚C (with a 67% likelihood). Using this carbon budget as an overarching benchmark, a new book released by the University of Technology Sydney (UTS) helps guide net-zero goals by laying out detailed, energy-related carbon budgets for 12 major industries.
The book, Achieving the Paris Climate Agreement Goals Part 2: Science-based Target Setting for the Finance Industry — Net-Zero Sectoral 1.5˚C Pathways for Real Economy Sector Springer Nature (2022) is designed as a continuation of this group’s 2019 first edition, which focused on country-specific energy pathways. Decarbonization pathways have been developed for countries, regions, and communities, but never before for industry sectors in a detailed way. While the book consists of 400 pages of dense methodologies and calculations, its topline message is clear; in the words of the lead author Sven Teske, “ We can limit global warming to 1.5˚C with the technology pathways we describe... I would call it an action plan to save the future for our children and their children."
The One Earth Climate Model (OECM) began as a research project supported by One Earth between the University of Technology Sydney, the German Aerospace Centre, and the University of Melbourne in 2017. They were tasked with developing a detailed 1.5˚C GHG trajectory for ten world regions without the continued use of fossil fuels or unproven technologies like carbon capture and storage. The results of the first model made it clear that it is still possible to limit warming to 1.5˚C with a rapid transition to 100% renewable energy sources. However, the model did not yet have the granularity the financial sector needed to guide and benchmark net-zero investments.
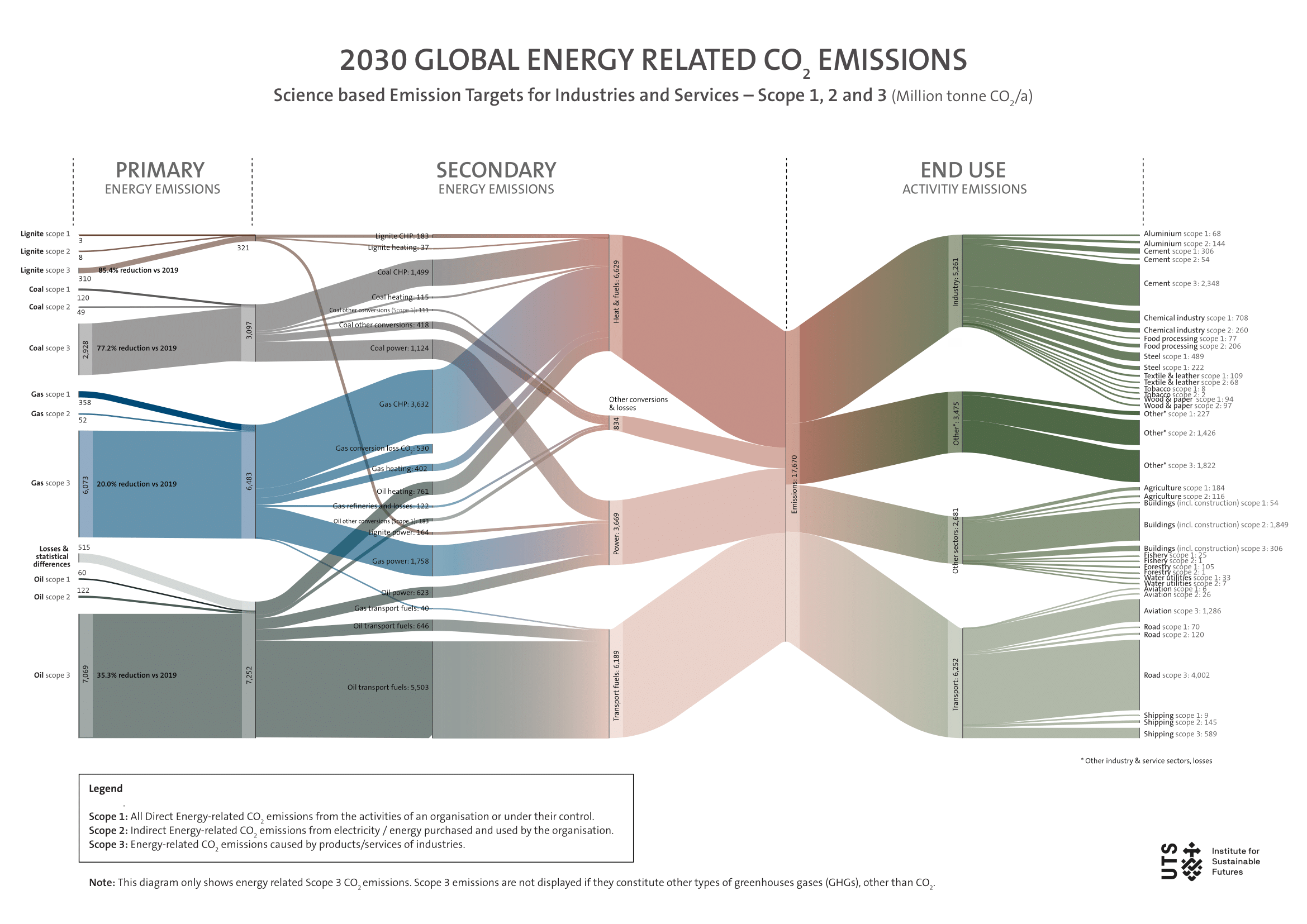
Global scope 3 emissions in 2030 under a 1.5C pathway, assigned by fuel (left hand side), division (primary, secondary and end-use) and industry (right-hand side). Source: Teske, S. et al. (2022).
This new model, OECM 2.0, was commissioned by the UN-convened Net-Zero Asset Owner Alliance (NZAOA), a group of 71 institutional investors with over $10 trillion USD in assets committed to developing net-zero emissions portfolios by 2050. The task was to “develop science-based sectoral decarbonization pathways and targets, broken down into scopes 1, 2, and 3, for industry sectors classified under the Global Industry Categorisation Standard (GICS)”. The GICS sectors covered include these 12 major industries:
- Aluminum
- Chemicals
- Cement
- Steel
- Textiles and leather industry
- Power utilities
- Gas utilities
- Agriculture
- Forestry
- Aviation and shipping industry
- Road transport
- Real estate and building industry
The new model improves on OECM 1.0 by merging three independent models into one interconnected MATLAB-based energy assessment model covering the entire global energy system. OECM 2.0 significantly increases the technical resolution of modeling efforts by incorporating energy demand and supply scenarios for each industrial sector based on sector-specific GDP projections, market forecasts, and material flows. This level of detail covering Scope 1, 2, and 3 emissions will allow the finance sector to develop emissions benchmarks and create time-bound targets to track their investments toward a net-zero goal.
3 Peer-reviewed papers
The publication of this book was preceded by three technical papers that cover different aspects of the model. The first paper, One Earth Climate Model—Integrated Energy Assessment Model to Develop Industry-Specific 1.5 °C Pathways with High Technical Resolution for the Finance Sector, uses the transportation sector to demonstrate how the model works. For all sectors, the model follows five steps to create a sector-specific decarbonization pathway.
Step 1: Calculate the future production in a specific sector using GDP projections, population projections, and industry-specific projections. In the case of the steel sector, for example, the “production” unit is measured in tons of steel per year. In the case of passenger road transportation, the “production” unit is the amount of passenger kilometers (pkm) driven per year. According to the model, passenger road travel is projected to stay largely the same despite an increase in global population largely due to the adoption of more rail infrastructure, which is projected to increase over three times.
Step 2: Calculate energy intensities (amount of energy per unit of production) over time by detailing technology type and projected gains in efficiency bespoke for every sector. For example, electric vehicles require about two-thirds less energy, mile-for-mile, than do internal combustion engines (ICE). In the table below, you can see the energy intensity of each vehicle type decreases over time as advances in efficiency are expected to come into effect.
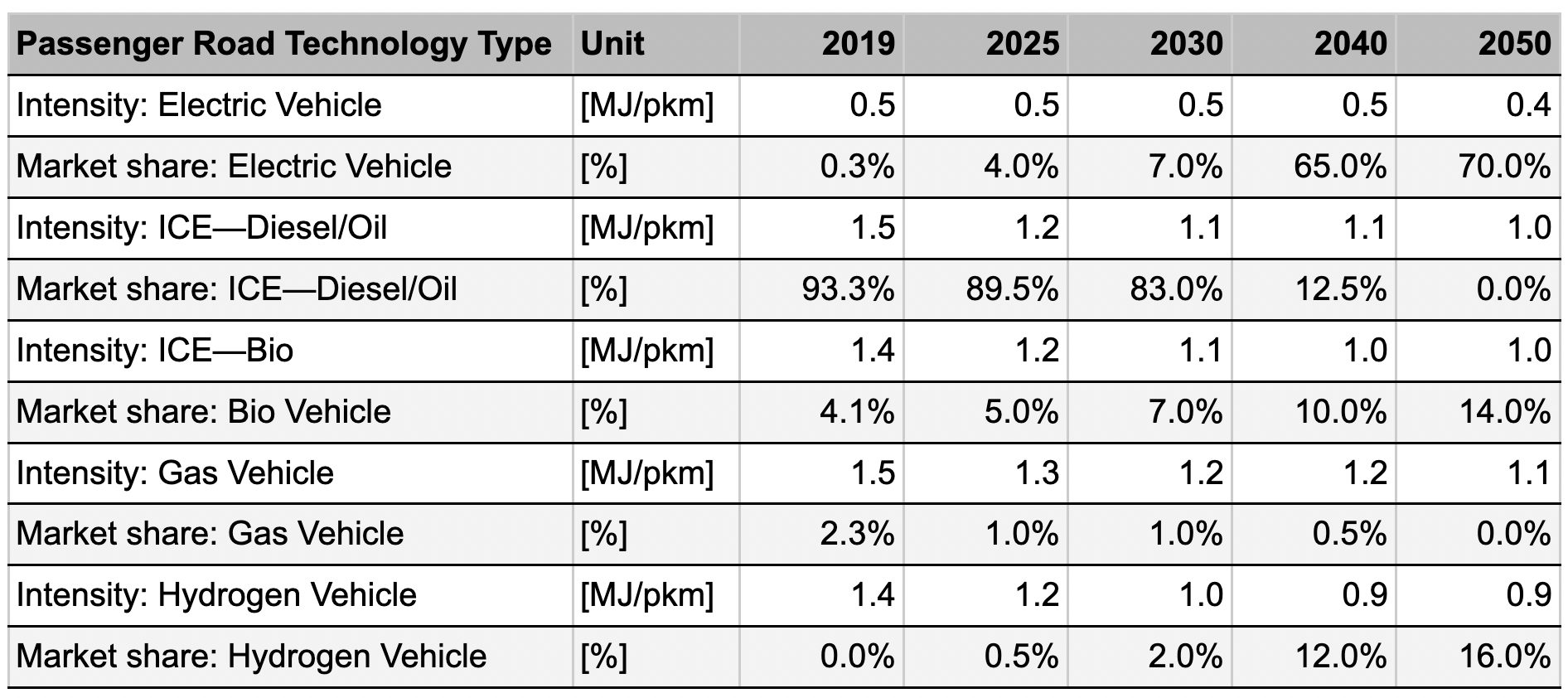
Projected market share and intensity of different passenger road technology types. Source: Teske, S. et al. (2022). Image Credit: Spencer R. Scott
Step 3: Calculate the energy demands from that sector by essentially multiplying the results of Step 1 and Step 2. Once you know the production volume (pkm per year) and the energy intensity per unit (MJ/pkm), you can calculate the total energy demand per year.
Step 4: Match the energy demands for different categories of electricity, heat, and fuel to the available energy supply. The global energy supply used in the model is essentially pre-fixed, as all sector demands are supplied by the same energy supply structure. In other words, The electricity demand for all sectors—residential, industry, and transport—is summed and is supplied as a whole. For passenger travel, the share of renewables is expected to increase from 4% market share in 2019 to 12% by 2030, 79% in 2040, and a full 100% in 2050.
Step 5: Calculate the sectoral emissions over time by matching the total energy demand with the available supply’s emissions intensity.
As you can see from this transportation example, more detailed input parameters, such as passenger-kilometers per year and the breakdown by vehicle type and transport mode, are required. Every sector requires this type of detailed input, but doing so enables sector-specific decarbonization pathways and ensures that the global economy can remain below its carbon budget.
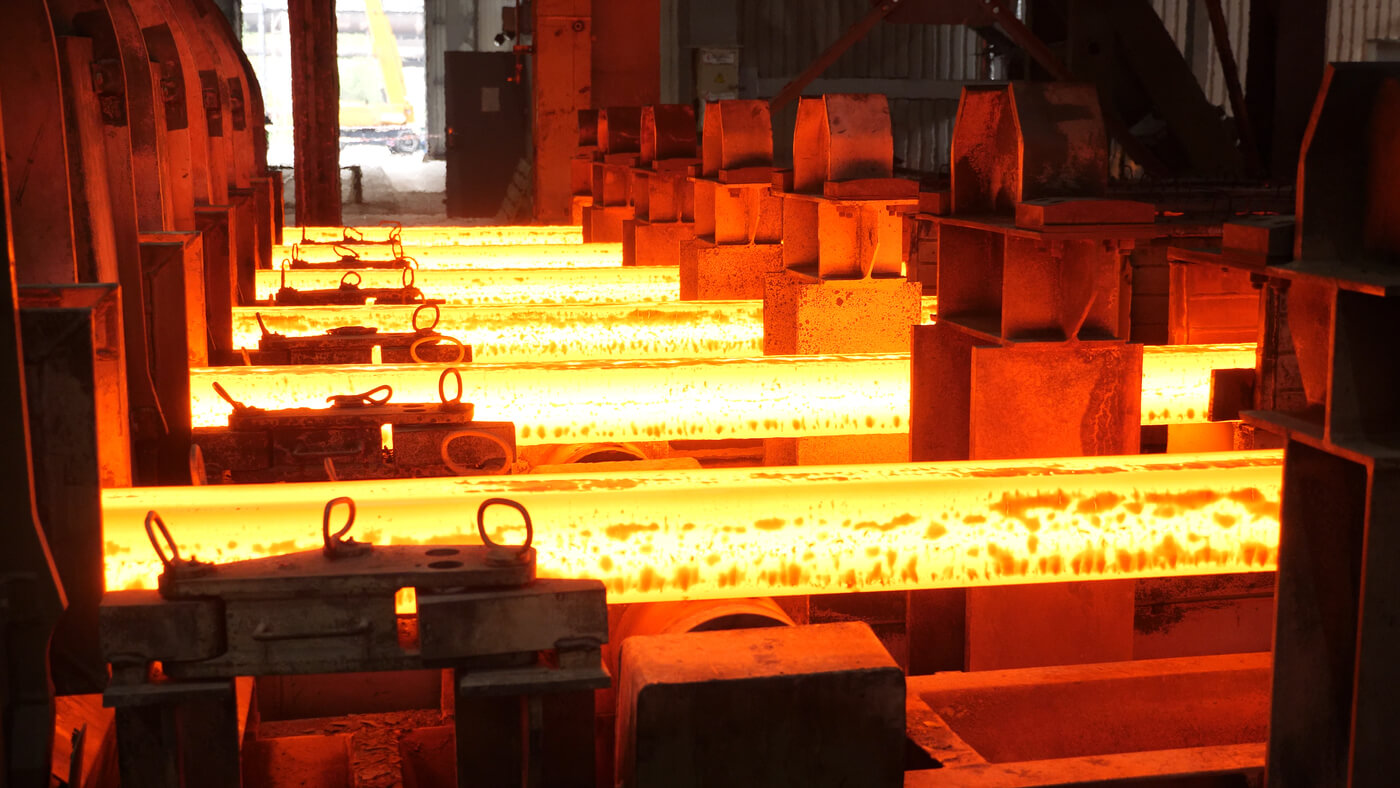
The second paper, 1.5 °C pathways for the Global Industry Classification (GICS) sectors chemicals, aluminum, and steel takes a closer look at a few hard-to-abate sectors to highlight how the model can incorporate different energy intensities based on temperature needs for specific industrial processes.
For each sector, the team created a bottom-up demand analysis by inputting sector-specific market projections and energy intensities across various variables within each sector. For example, the energy demand of steel is driven by annual production in tons of steel as well as the availability of secondary steel and the need to mine virgin iron ore.
Recycling secondary steel requires a lower temperature (and up to 74% less energy) than creating steel from virgin ore; these different temperatures require different energy supplies and, therefore have different energy intensities, which the final energy demand is calculated by. For all sectors, the calculation of process heat was broken into four temperature buckets: low (<100˚C), medium low (100-500˚C), medium high (500-1000˚C), and high (>1000˚C) with low heat being met by electricity powered heat pumps and high heats relying on green hydrogen or other renewable fuels.
The paper outlines in detail the expansive set of input data required to create bespoke energy demand projections for each sector. For example, about 72% of the aluminum industry requires high-temperature heat while only 57% of the steel industry requires high-temperature heat. Doing so demonstrates how ambitious the energy efficiencies for all three industries must be and how a large increase in aluminum and steel recycling is necessary. Even so, the electricity demand for both the chemical and steel industries is projected to double, while the aluminum industry is set to rise modestly.
Modeling the specifics of energy demand helps create a more realistic test for how our global electricity grids can transition to 100% renewable energy while meeting all demand and staying under the IPCC’s carbon budget of 400 Gt. These models will help guide where money needs to be invested to have the necessary renewable technologies poised to meet that demand.
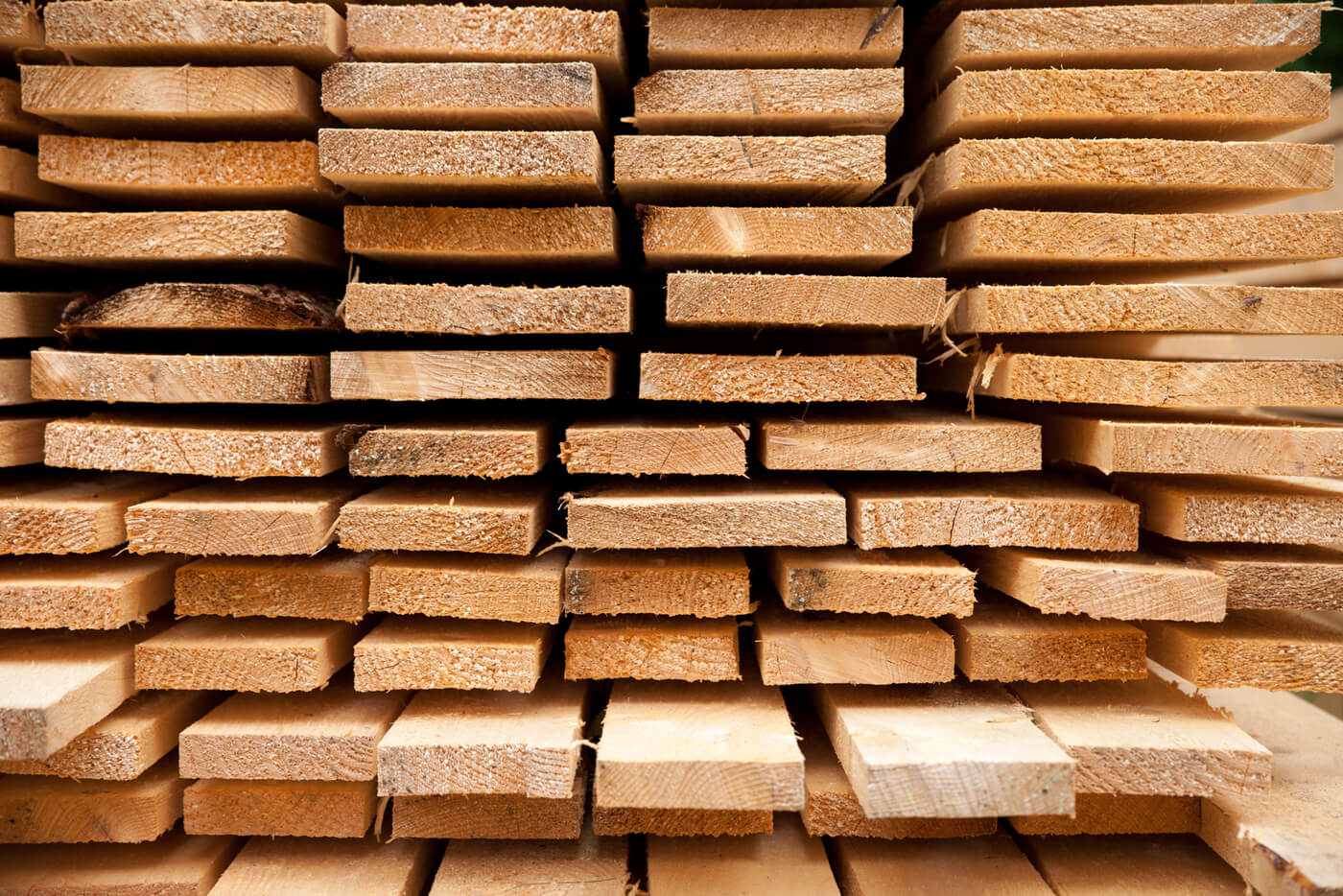
The third paper, Global sector-specific Scope 1, 2, and 3 analyses for setting net-zero targets: agriculture, forestry, and processing harvested products, introduces a new methodology to assign Scope 1-3 energy emissions across the major industry sectors and uses the Agriculture & Food and Forestry & Wood Products sectors as examples.
In order to set net-zero targets, disaggregated information on the Scope 1, 2, and 3 emissions of industry sectors is needed, and currently, the International Energy Alliance (IEA) does not provide that data. This paper set out to provide that analysis. The paper used the Greenhouse Gas Protocol in defining the three scopes:
- Scope 1 emissions occurring directly from sources owned or controlled by a company;
- Scope 2 emissions indirectly resulting from the generation of purchased energy;
- Scope 3 emissions indirectly resulting from the “value chain” of the reporting company and not included in Scope 2.
Scope 3 emissions are notoriously difficult to disentangle, which leads to justified concerns of double-counting. However, this research delivers a groundbreaking methodology that clearly separates Scope 3 emissions to avoid double counting by tracking emissions from their primary source, through their secondary industry category, and onto their final end-use sector. By using the known total primary energy emissions, all scopes could be capped so that double-counting is impossible. So for each sector, Scope 3 only includes sector-specific emissions. For example, traveling and commuting are reported under the Transport sector, and the lease of buildings are reported under the Buildings sector. This analysis demonstrates that achieving net-zero emissions under the Paris Agreement for a specific industry sector “Requires all its business activities with other sectors to also commit to 1.5˚C net-zero emissions targets.”
This paper laid out robust decarbonization pathways across all Scopes for the Agriculture and Forestry sectors. For scopes 1 and 2 that consists of increased energy efficiency, a shift to a renewable energy supply, and the replacement of fossil-fuel-driven machinery with electric and bio-fuel vehicles.
Although the forestry sector has a clear pathway to net-zero and even into net-negative emissions, the agriculture sector is not expected to fully decarbonize its non-energy GHGs by 2050. Due to an increasing and developing global population, there is a need for crop intensification, agricultural expansion, and an expected increase in meat-based diets. Together these will be responsible for substantial emissions from fertilizer applications and methane emissions from meat production. This highlights the need for a suite of technologies to reduce these emissions or a shift in diets away from high-meat consumption. In either case, these results will help institutional investors make decisions on where private capital is crucially needed as the world transitions to net-zero emissions.
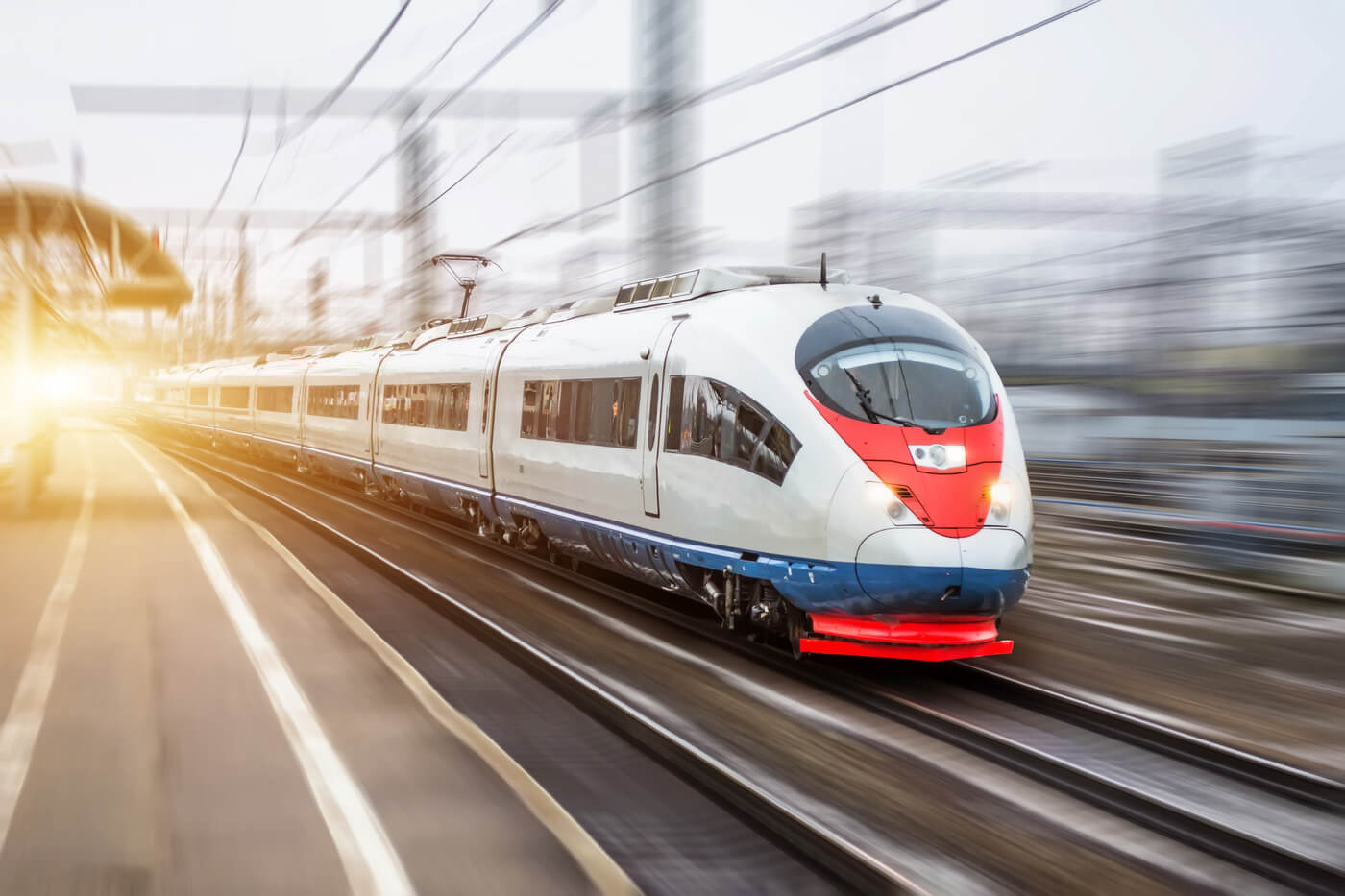
The future of 100RE modeling
The OECM 2.0 model is one of the most advanced decarbonization models ever undertaken, but it is part of a larger and growing field of scientific efforts to develop optimized transition pathways to 100% clean, renewable energy for all uses, known as 100RE. A new paper, also co-authored by Sven Teske, On the History and Future of 100% Renewable Energy Systems Research delves into the history of these modeling efforts. Once met with strong skepticism, 100RE models have grown in credibility as solar and wind costs have plummeted, and recent papers have worked on the challenges of smart grids and energy storage. In fact, “the main conclusion of most of these studies is that 100% renewable is feasible worldwide at low cost,” charting a course forward toward a fossil fuel free future. Modelers play a vital role in informing decision makers across governments, NGOs, businesses, and financial institutions. Trillions of dollars will be spent in the coming decade to get the world on track to 1.5°C, and these models help to show how we can deploy this capital to achieve the greatest public good.
Explore the One Earth Solutions Framework